Organoid Intelligence and Cellular Communication Pathways
- Lawrence Cummins
- Nov 23, 2024
- 6 min read
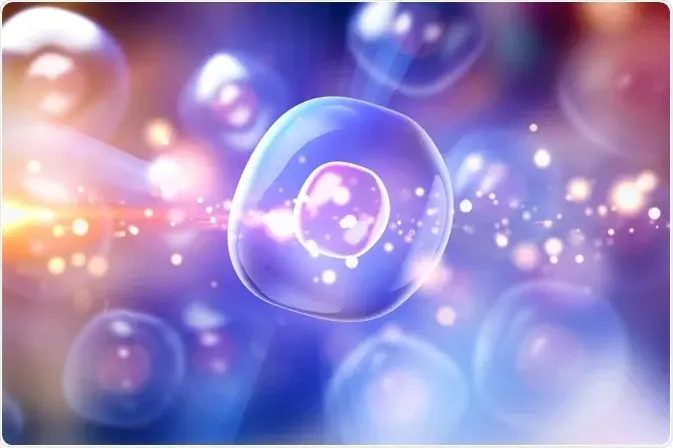
Cellular signaling pathways play a critical role in shaping the cognitive functions of organoid
intelligence, particularly in brain organoids that model aspects of human neural development and functionality.
Here are several ways in which these pathways contribute to cognitive functions:
Cellular Communication:
Organoid intelligence, the integration of organoid biology and artificial intelligence, represents a pioneering domain of research that sheds light on the intricate mechanisms of cellular communication and signaling pathways. Organoids, which are three-dimensional organ-like structures derived from stem cells, mimic the architecture and functionality of specific tissues. This unique characteristic allows researchers to study complex cell interactions in a controlled environment, shedding light on how cellular signaling influences development, homeostasis, and disease processes.
Cellular communication is predominantly mediated through signaling pathways that involve interactions among various biomolecules, such as hormones, proteins, and neurotransmitters. These pathways, including the Notch, Wnt, and MAPK signaling cascades, orchestrate fundamental biological processes such as cell differentiation, proliferation, and apoptosis. By utilizing organoid models, scientists can elucidate the dynamics of these signaling networks, gaining insights into their contributions to tissue resilience and pathology.
The Notch signaling pathway regulates cell differentiation, proliferation, and apoptosis. This pathway is characterized by direct cell-to-cell communication, where membrane-bound Notch ligands on one cell interact with Notch receptors on an adjacent cell. This interaction triggers a series of intramolecular cleavages, ultimately releasing the Notch intracellular domain (NICD), which translocates to the nucleus to regulate gene expression.
In organoid cultures, Notch signaling is crucial for maintaining the balance between stem cell renewal and differentiation, permitting the formation of diverse cell types essential for organoid functionality. The Wnt signaling pathway, another critical player, regulates cell fate, proliferation, and migration during embryonic development.
Wnt proteins are secreted glycoproteins that bind to Frizzled receptors on target cells, stabilizing β-catenin in the cytoplasm. When active, β-catenin translocates to the nucleus, prompting the expression of target genes involved in cell proliferation and differentiation. In the context of organoids, Wnt signaling activation is indispensable for establishing epithelial structures and maintaining the functionality of various organ systems, such as the intestine and brain.
The Mitogen-Activated Protein Kinase (MAPK) pathway is a crucial signaling cascade that transmits extracellular signals to elicit cellular responses such as growth, differentiation, and stress. Various stimuli, including growth factors, activate the MAPK pathway, which leads to a series of phosphorylation events involving MAPK kinases. This ultimately activates effector proteins that regulate gene expression and cellular behavior.
In organoids, MAPK signaling is involved in processes such as tissue regeneration and responses to external stimuli, thereby contributing to the dynamic adaptability of organoid cultures. The exploration of organoid intelligence reveals the sophistication of cellular communication through signaling pathways like Notch, Wnt, and MAPK. Understanding these pathways enhances our knowledge of organoid biology and facilitates advancements in therapeutic strategies and personalized medicine.
As research in this area progresses, the potential applications of organoid intelligence in regenerative and translational medicine continue to expand, heralding a new era in biological research and its clinical implications. The intersection of organoid technology and AI further enhances our understanding of these signaling pathways by enabling the analysis of vast datasets, ultimately leading to predictive models of cell behavior. As this field progresses, organoid intelligence holds immense potential for advancing personalized medicine, drug development, and regenerative therapies, transforming our approach to complex biological challenges.
Neuronal Differentiation and Maturation:
Signaling pathways involving growth factors (like BDNF and NGF) and neurotransmitters are crucial for differentiating stem cells into neurons and glial cells. These processes influence the development of various neuronal subtypes that contribute to specific cognitive functions.
Brain-derived neurotrophic Factor (BDNF) and Nerve Growth Factor (NGF) are pivotal neurotrophic factors in neurobiology and emerging fields such as organoid intelligence.
BDNF is primarily involved in the maintenance, survival, and growth of neurons, playing a significant role in synaptic plasticity and cognitive functions such as learning and memory. Its influence on neurogenesis and synaptic connectivity makes it a crucial player in healthy brain function and neural repair mechanisms.
Nerve Growth Factor (NGF) is essential for the survival and differentiation of specific neurons, particularly those in the peripheral nervous system. It facilitates the growth of neuronal processes and affects neuroplasticity, influencing various neurological conditions and responses to neural injuries.
Synaptic Plasticity:
Synaptic plasticity, the ability of synapses to strengthen or weaken over time, is fundamental to learning and memory. This dynamic process is influenced by several molecular pathways that regulate neuron function and connectivity. Among these, N-methyl-D-aspartate receptors (NMDARs), protein kinase C (PKC), mitogen-activated protein kinases (MAPK), and the Mre11-Rad50-Nbs1 (MRN) complex have emerged as critical players in orchestrating synaptic adjustments, particularly within the framework of organoid intelligence. NMDARs are specialized glutamate receptors that play a pivotal role in synaptic plasticity.
They are unique in their requirement for ligand binding and postsynaptic depolarization. This allows calcium ions to flow into the neuron, triggering intracellular signaling cascades. This calcium influx is essential for inducing long-term potentiation (LTP), a process that strengthens synaptic connections and is fundamental for learning. In the context of organoids, miniature, simplified versions of organs created from stem cells, NMDARs enable the development of functioning neural networks, simulating aspects of human brain activity.
Protein kinase C (PKC) acts downstream of NMDAR activation and modulates synaptic plasticity. PKC influences various neuronal signaling pathways, including those that regulate neurotransmitter release and receptor sensitivity. By playing a regulatory role in synaptic restructuring, PKC supports both LTP and long-term depression (LTD), ensuring a balanced neural response that is critical for adaptability and memory formation. MAPK pathways, which include extracellular signal-regulated kinases (ERK), are also crucial in synaptic plasticity.
They respond to growth factors, neurotransmitters, and other signals to influence gene expression and protein synthesis, affecting synaptic structure and function over longer time scales. This pathway’s integration into organoids offers insights into how external stimuli can alter network properties, enhancing computational capabilities reminiscent of cognitive function. The MRN complex, primarily known for its role in DNA repair, is also implicated in synaptic plasticity.
Neurogenesis:
Cellular signaling also regulates neurogenesis (the formation of new neurons), particularly in regions analogous to the hippocampus in organoids. This process is essential for memory formation and cognitive flexibility.
Neurogenesis cellular signaling refers to the complex processes regulating the generation of new neurons from neural stem cells, primarily in the brain. This phenomenon is crucial for neuroplasticity, enabling adaptive responses to environmental stimuli and playing a significant role in learning and memory. The signaling pathways involved in neurogenesis include various growth factors, neurotransmitters, and neurotrophic factors, which collectively facilitate the proliferation, differentiation, and maturation of neural progenitor cells.
Understanding neurogenesis is pivotal in the context of Organoid Intelligence (OI), which seeks to replicate brain functionalities using organoids, miniaturized and simplified versions of organs, is pivotal. Organoids, particularly cerebral organoids, can model various aspects of human brain development and functioning, leading to groundbreaking advances in artificial intelligence (AI). By incorporating principles of neurogenesis, researchers can enhance the performance of organoids in processing information, akin to biological brains.
Cellular signaling mechanisms that govern neurogenesis can inform AI algorithms, as they involve adaptive learning and dynamic responses to stimuli. As OI evolves, leveraging insights from neurogenesis may lead to developing more sophisticated cognitive functions in AI systems, potentially bridging the gap between biological intelligence and artificial cognition. The study of neurogenesis cellular signaling is integral to actualizing the potential of Organoid Intelligence in artificial intelligence applications.
Disease Modeling:
Organoid intelligence represents a significant advancement in disease modeling, harnessing the potential of human-derived organoids to simulate complex biological systems. Organoids are miniaturized, three-dimensional structures derived from stem cells that replicate the architecture and functionality of specific organs. Their application in disease modeling is particularly transformative for understanding pathophysiological processes and testing therapeutic interventions.
In disease modeling, organoid intelligence allows researchers to observe cellular interactions and disease progression in a controlled environment, offering insights that traditional two-dimensional cell cultures cannot provide. For instance, organoids have been used to model cancer, neurodegenerative, and infectious diseases, providing a more accurate representation of human physiology and disease mechanisms.
This capability enables identifying disease-specific biomarkers, elucidating drug responses, and exploring genetic modifications. The integration of advanced technologies, such as single-cell sequencing and high-resolution imaging, further enhances the utility of organoids in disease modeling. As researchers continue to refine these models, organoid intelligence promises to bridge the gap between laboratory findings and clinical applications, ultimately advancing precision medicine and improving treatment outcomes for patients afflicted with various diseases. The ongoing evolution of this field underscores its potential to revolutionize biomedical research and therapeutic development.
Comments